Aleenah Ansari
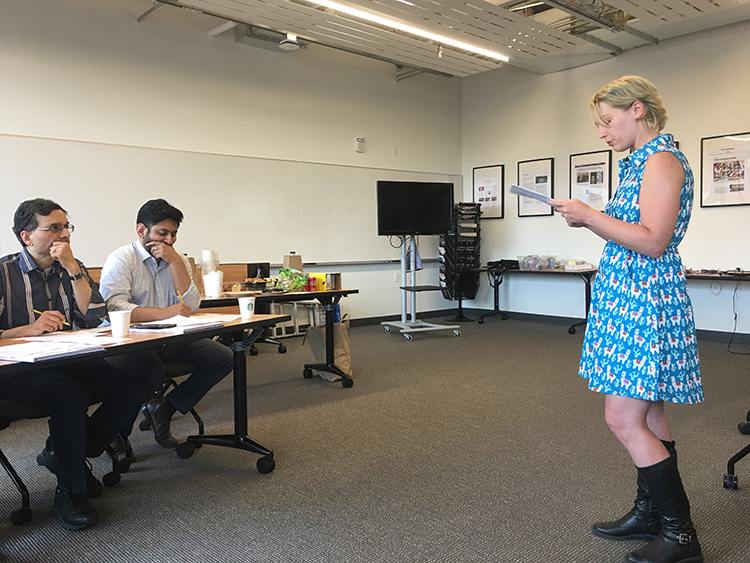
On May 29, 2019, graduate students were challenged in a competition at the CNT to explain their research in 90 seconds using only the thousand most common words in the English language.
Researchers are often asked to formulate questions, design experiments, and solve problems while considering limitations in data, funding, or other resources. For the graduate student researchers who participated in the Ten Hundred Words of Science Competition held on May 29 at the Center for Neurotechnology (CNT), their only constraint was the type of words they could use to explain their research.
Ishan Dasgupta, a post-doctoral researcher at the CNT and University of Washington’s (UW) Department of Philosophy and judge for this event. saw this competition as an opportunity for researchers to tell a story about their work and its impact. He said that scientists are responsible for communicating the goal of their research and its impact, especially when talking to the general public and researchers in other fields.
“A lot of science is a story. If you can convey it, it has great potential in making a difference,” Dasgupta said. “In our center, we often use examples of success stories from people who have been touched by these technologies.”
During the competition, each graduate student had 90 seconds to describe their research in an oral presentation using only the thousand most common words in the English language. For example, they could describe their research as taking place in “computer buildings” (data centers) or the impact of their work on the “bags of stuff inside a person” (human organs). Afterwards, each participant had 60 seconds to provide a more technical explanation of their research so the audience and judges could compare both explanations.
“You want to be able to explain your research whether you’re with your grandmother at a dinner, or if you’re on a plane next to a governor and want to advocate for your research,” said Claire Rusch, a fourth-year PhD student in the UW Department of Biology and outreach officer for the CNT’s Student Leadership Council. “It’s helpful for researchers to have a speech that they can give to everyone and convey the importance of their work.”
Hosting the competition this year was an outreach goal for Rusch. She saw it as a way to augment K-12 education outreach at the CNT, which focuses on educating students about neuroscience, neural engineering, and developments in brain-computer interfaces. These outreach efforts occur through events and programs such as the Brain Awareness Week for K-12 students, the Young Scholars Program- REACH for high school students in the Greater Seattle Area, and partnerships between the CNT and the UW DO-IT program.
More broadly, outreach events like the Ten Hundred Words Competition can help the public learn about a broad spectrum of research that is done today.
“Science impacts people, whether it’s health, environment or climate change,” Rusch said. “Most of the things we do are paid with taxpayer money, and [taxpayers] deserve to hear how we are using their money.”
Melanie Anderson, a graduate student in the UW Department of Mechanical Engineering presented her research about the Smellicopter, an autonomous palm-sized nanodrone. It tracks odors with antennae that are removed from a moth and attached to an electrical circuit. Long-term, these antennae could be modified to smell a variety of different substances, such as chemicals and odors from explosives.
“Right now, my tiny smelling flying computer will only respond to smells that the
[the moth] is interested in,” Anderson said when giving the simplified explanation of her research. “It is possible to change what the animal can smell so my tiny smelling flying computer can smell smoke from fires and sense other things in the air which might hurt people.”
Another participant was Nassima Bouzid, a graduate student in the UW Department of Biology. Rusch read Bouzid’s simplified, written explanation first, which summarized Bouzid’s research on the physical appearance of animals living in “cold high places and warm low places.” In the technical explanation of her research, Bouzid said that her work focused on the physical appearance of Western fence lizards and how it varies based on the lizard’s level of activity and the climate in which they live.
Yoni Browning, a graduate student in the UW Department of Biology, explained the way that the brain forms new memories, which is the focus of his research in the UW Buffalo Lab.
“In order to form a new memory, the brain must keep track of what is happening to [the monkey],” Rusch said when reading Browning’s simplified explanation, which described his research about how monkeys visualize space and memory. “After all, if the brain doesn’t know about what is going on now, how can it be remembered later?”
Lastly, Aji John, a graduate student in the UW Buckley Lab, described that “hot days are not that hot when you’re under old trees.” John went on to explain that trees in old-growth forests have lived between 500 and 2,000 years without being logged, and their canopies can regulate temperatures and protect native species of wildlife and plants in the area.
“These trees have the capacity to buffer macro-climatic conditions,” John said in his technical explanation. “I study the microclimates in these old-growth forests and how they relate to macroclimatic conditions.”
The judging panel included Dasgupta; Andreas Schönau, a post-doctoral research associate at the CNT; and Mak Agashe, the CEO of Pifocal who represented an industry perspective. The three judges evaluated each simplified and technical presentation based on how well it communicated the concept, context and potential impact of the presenter’s research in society.
After the judges deliberated, Anderson was awarded a copy of the book, Thing Explainer: Complicated Stuff in Simple Words by Randall Munroe, for best technical explanation, and Browning won a copy for best simplified explanation of his own research. Anderson also won a UW Bookstore gift card for best overall presentation.
Rusch hopes that this event encouraged conversation among scientists in disciplines ranging from mechanical engineering to neuroscience. Dasgupta also saw the Ten Hundred Words Competition as an opportunity for the public to learn more about new research and provide feedback.
“It’s not just that we have an obligation [to make our work understandable], but understanding what the public has to say [about our research] improves science as a whole,” Dasgupta said.
Are you a research scientist? Try writing about your own research with the ten hundred most common words in the English language using the Up-Goer Five Text Editor.
Some submissions from the Ten Hundreds Words Competition are available below.
Local adaptation in Western Fence Lizards along an elevation gradient –Nassima Bouzid
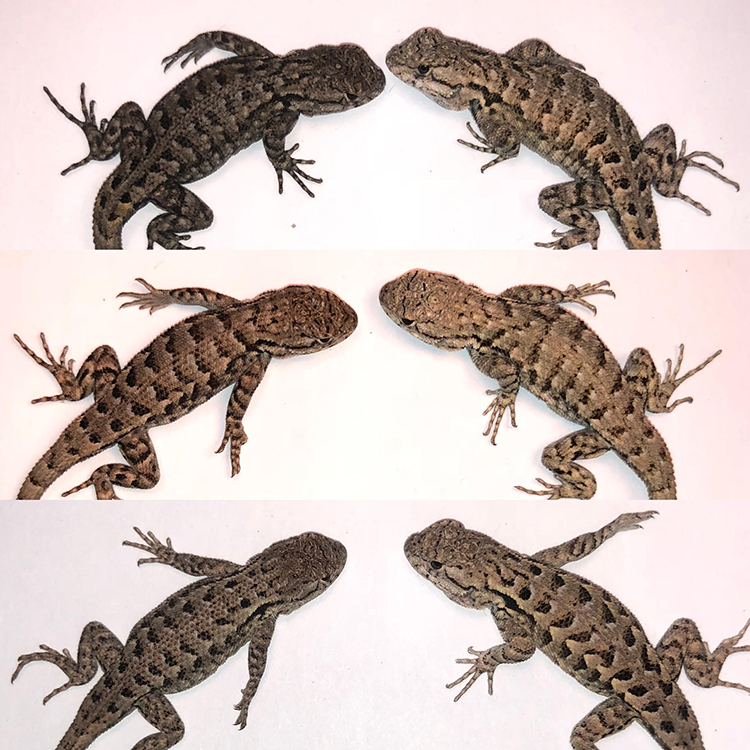
Simplified Explanation
I study animals that live on rocks and use the sun to grow. In cold places, where there is little time in the sun, it is hard for these animals to grow. Two families of animals live in two places: one low place that is warm and one high place that is cold. In the warm, low place the animals are small and light-colored and in the cold, high place the animals are big and dark-colored, but animals from both places are in the same family! Why do they look different? In the cold, high place the animals hide for a long time because of ice-rain on the ground–so why are they bigger? I raised babies from both places to see if they were different because of blood or because of place. I found that animals from the hot, low place spent less time outside and more time hiding, while animals from the cold, high place spent more time outside and less time hiding. So, even though it is cold in the high place the animals spend more time outside to grow, and they are not cold because their black color helps them get warm faster. Even though it is warm in the low place, the animals hide because they are afraid of things that will eat them. So, the animals in the warm, low place have less time to grow and they are smaller. I found that whether the animals hide or stay outside is because of blood, and they look different because of whether they hide or stay outside.
Technical Explanation
Western Fence Lizards (Sceloporus occidentalis) from an elevation gradient in Yosemite National Park, California exhibit a phenotypic cline. Phenotypes vary from small, light-colored individuals at low elevations to large, melanistic individuals at high elevations. High elevation phenotypes may reflect local adaptation to cooler conditions and limited activity periods; melanistic coloration and larger body sizes facilitate heat gain and heat retention, respectively, which may enable individuals to extend activity periods in cool environments. To determine whether divergent phenotypes were produced by genetic differentiation or by phenotypic plasticity, and whether phenotypes are locally adapted, we reared hatchling lizards in a lab reciprocal transplant experiment. We found evidence for locally adapted behavioral strategies that may drive phenotypic divergence. High elevation lizards, regardless of environment, maximized activity while low elevation lizards, regardless of environment, were more conservative with activity. High elevation lizards grew faster because they spent more time feeding and basking. In cooler environments, more melanistic coloration may be an accessory to extended activity. Low elevation lizards grew more slowly because they spent less time feeding and basking. Predation pressure is higher in warmer, low elevation environments, meaning that conservative activity may be adaptive.
How the brain forms new memories – Yoni Browning
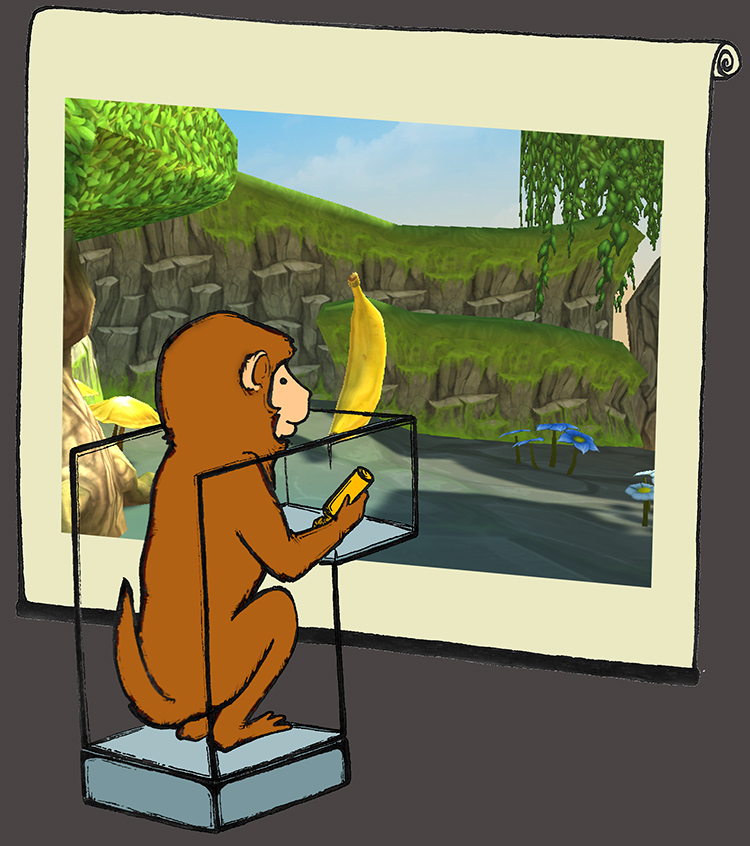
Illustration by Sierra Schleufer
Simplified Explanation
We know that there are parts of the brain that people need to make new memories, but we don’t know very much about how these brain areas work. Part of the problem is that we do not know how single brain cells act in these memory-making parts of the brain. In order to form a new memory, the brain must keep track of what is happening to an animal. After all, if the brain doesn’t know about what is going on now, how can it be remembered later? Because of this, we expect brain areas that make new memories should keep track of what is happening to an animal as it happens. We trained some animals to drive around in a computer game. In this computer game we have the animals do the same things many times, and look at what each brain cell does each time they do it. Sure enough, we see brain cells that act the same way each time the animal does the same thing. Most often, this means that brain cells get excited each time the animal drives past the same spot in the computer game. Other times, brain cells are excited at key moments in the game. Even though our work can only look at brain cells one at a time, the fact that each cell keeps track of a little piece of what the animal is doing suggests that many of these cells work together to track all of what is happening to the animal. This is exciting, because it may tell us how the cells in the brain area that makes new memories might work together to help this brain area do its job.
Technical Explanation
The hippocampus and surrounding cortex are necessary for the formation of episodic memories, that is, memories of remembered events. However, it is unclear how activity of individual neurons supports mnemonic function. One possibility that the ongoing activity in the hippocampus tracks features of the animal’s experience, and the hippocampus supports memory by linking salient events into a continuous, unitary episode. To characterize task-relevant activity in the hippocampus, we trained monkeys to navigate a maze virtual reality. Our task has a highly repeatable structure, allowing us to study the activity of individual neurons as the monkey performs a consistent task. We have identified neurons with responses to the monkey’s position in virtual space. The spatial preferences of these neurons tend to cluster around the key events in the maze (start point, goal locations, etc.), emphasizing the important role that task structure plays in shaping hippocampal activity. Further, these data are congruent with the idea the hippocampus tracks consistent task features.
A tiny smelling flying computer | Melanie Anderson
Simplified Explanation
It turns out that tiny flying animals, like the annoying ones that fly around lights at night, smell very well. They smell many times better than anything that people have made using little computer parts. If we can make a tiny flying computer that can smell things, then we can use it to approach problems such as smelling smoke from fires in the woods quickly before the fire grows too big, or, if the ground shakes and people are stuck under fallen down houses, we can send the little flying smelling computers out, like little flying search dogs, to quickly find the hurt people. We can also use it to learn more about how the tiny flying animals smell so much better than us and fly around so well. But humans haven’t made anything that is small enough, can smell well enough, and responds quick enough to go on a tiny flying computer. So I have cut off the smelling part from the tiny flying animals and put it together with small computer parts to make a tiny smelling flying computer! When you cut the smelling parts from the tiny flying animal, the smelling parts will live and keep on smelling for many hours all by themselves. You can put that part onto the tiny flying computer, and now the computer can sense when the part smells something and can fly in the direction of the smell. This way, the computer can find where the smell is coming from. Right now, my tiny smelling flying computer will only respond to smells that the animal is interested in, but it is possible to change what the animal can smell so that my tiny smelling flying computer can smell smoke from fires and sense other things in the air which might hurt people.
Technical Explanation
My project is the Smellicopter, which is a bio-hybrid autonomous odor localizing nanodrone. Commercially available chemical and odor sensors are not small enough or have fast enough response times to fly on a small drone, so I turned to nature. Moths rely on their sense of smell for finding mates and finding food, and they do so with astounding accuracy. So I have taken the antenna from the moth and attached it to a small electrical circuit which can measure the signal from the antenna when it senses odors. Using this, I’m able to program a drone that fits in the palm of my hand to use these signals to find and track odors to their source. Right now, my drone only smells scents that the moth is interested in, but we are working on genetically modifying the moth to be more sensitive to other odors, such as dangerous chemicals and odors from explosives.